Abstract
Surface application of urea-containing fertilizers can result in significant losses of nitrogen (N) in the form of ammonia. Various soil and environmental factors such as soil texture, pH, cation exchange capacity (CEC), soil moisture, relative humidity and windspeed at the time of and directly following application affect ammonia volatilization. The objectives of this paper are to review soil properties and environmental factors that affect ammonia loss (AL) from surface applied urea and discuss management strategies to reduce AL from applied urea-based fertilizer. Because of the many variables that affect AL and their interactions, the prediction of AL from urea is not easy. However, by simply knowing these factors and how they affect AL one can avoid or adjust the application of urea when conditions favor AL.
Introduction
Urea, anhydrous ammonia, and nitrogen (N) solutions are widely used sources of N in the U.S. Urea is the world’s most used solid N fertilizer. In Wisconsin, consumption of urea was reported to be 342,000 tons from July 2022 to June 2023, which accounts for more than 45% of total N fertilizer consumption in the state. The use of urea ammonium nitrate (UAN) (28-, and 32%) was reported to be around 116,000 and 177,000 tons, respectively, over the same period (DATCP, 2024). These fertilizers, like all ammonium-based fertilizers, have the potential to lose N through ammonia volatilization, hereafter called ammonia loss (AL). Here we focus on AL from urea-based solid and liquid fertilizers.
Reactions in the soil
When urea-containing fertilizers are applied to soil, they quickly hydrolyze. Hydrolysis is a biological process that is catalyzed by the enzyme urease. This process produces ammonium carbonate, which then breaks down into carbon dioxide and ammonia as follows:
Equation 1: (NH2)2CO + 2H2O → (NH4)2CO3
Urea + Water → Ammonium Carbonate
Equation 2: (NH4)2CO3 + 2H+ → 2NH4+ + CO2 + H2O
Ammonium Carbonate + Hydrogen → Ammonium + Carbon Dioxide (gas) + Water
Equation 3: NH4+ + OH– ↔ NH3 + H2O
Ammonium + Hydroxyl ↔ Ammonia + Water
Factors that Affect Ammonia Volatilization
Ammonia volatilization contributes significantly to N loss from agricultural fields. Pan et al (2016) showed that globally, AL from applied N ranged from 0.9 to 64% (average of 18%). In a meta-analysis, Silva et al. (2017) also found significant variability in AL, with estimates ranging from 2.9 to 40% (average of 31%). A Wisconsin study reported AL between 1-19% from surface-applied urea, UAN, and ammonium nitrate in corn and grass pasture systems (Table 1) (Oberle and Bundy, 1988).
Table 1. Ammonia loss and yield from surface-applied fertilizers in corn and grass pasture at Lancaster, WI. Adapted from Oberle and Bundy (1988).
Corn | ||
N Source | Ammonia Loss (%) | Relative Yield (%) |
None | – | 63 |
Urea | 16 | 92 |
UAN (28%) | 12 | 95 |
Ammonium nitrate | 2 | 100 |
Pasture | ||
N Source | Ammonia Loss (%) | Relative Yield (%) |
None | – | 57 |
Urea | 19 | 84 |
Ammonium nitrate |
1 | 100 |
When incorporated, AL can be reduced to negligible levels as shown in a study by Rochette et al (2013). In this study, AL was 50% with surface application and decreased at a rate of 7% per centimeter of increased tillage depth. Thus, at incorporation depths of > 7.5 centimeters (~ 3 inches), AL was negligible. However, mechanical incorporation might not always be practical or desired as in the case of no-till.
Many other factors such as soil texture, surface residue, soil moisture, humidity, pH, windspeed, and ambient and soil temperatures impact the degree of AL from surface-applied urea. In the next section, we discuss soil, environmental, and management factors that control AL following surface application of urea.
Soil Properties and Ammonia Volatilization
Cation Exchange Capacity, Soil pH, and Temperature
When applied to soil, urea can increase the pH at the site of contact with the soil. This increase in pH is caused by urea hydrolysis during which hydrogen (H+) is consumed (Equation 2). The pH affects the form of ammoniacal N present in soil (Table 2). Soils with a higher pH have a greater potential for AL because more of the ammoniacal N is present in the form of ammonia relative to soil with a lower pH (Figure 1). Jones et al. (2007) noted that dissolved NH3, or ammonia in soil solution, increases significantly when the soil pH rises above 7.5, thereby increasing the potential for AL. The buffering capacity of the soil, or the soil’s ability to resist changes in pH, also affects AL. A poorly buffered soil will see a more rapid increase in pH and is thus more susceptible to AL. Soils that are poorly buffered against changes in pH, such as sandy soils with low cation exchange capacity (CEC) have a higher risk for AL in comparison to soil with a high clay content and CEC.
Table 2. Effect of soil pH on ammonia and ammonium. Adapted from Bundy (2001).
Ammoniacal N (%) | ||
Soil pH | Ammonia | Ammonium |
6 | 0.058 | 99.94 |
7 | 0.57 | 99.43 |
8 | 5.4 | 94.6 |
9 | 36.5 | 63.5 |
Microbial processes and activity are very temperature-dependent. An increase in soil temperature results in an increase in the enzymatic activity of urease. Consequently, urea hydrolysis is more rapid at higher temperatures. High temperatures also result in higher AL because they increase the soil concentrations of dissolved NH3 (Figure 1).
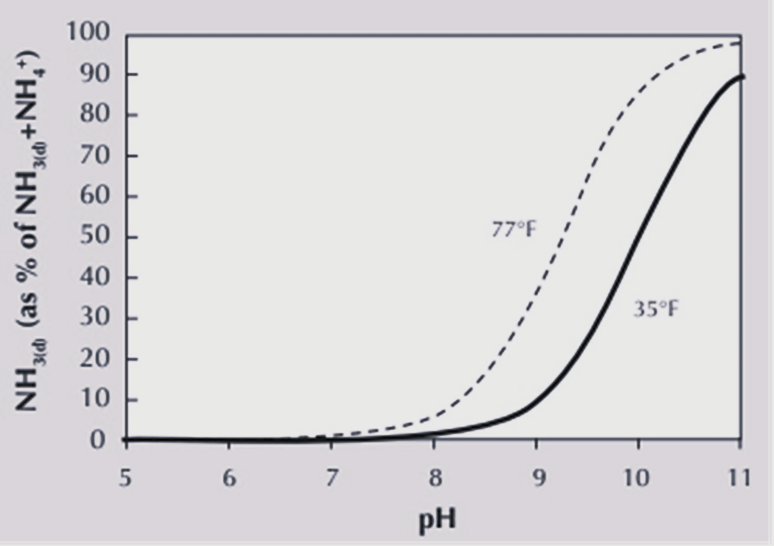
Figure 1. Effect of pH and temperature on dissolved ammonia (NH3(d)) and a percentage of total NH3(d) and NH4+ in soil (Jones at al., 2007).
Research at the University of Minnesota has shown that at a soil temperature of 60°F, 2% of added N is lost in AL 4 days after urea application while at 75°F, AL increased to 4% of applied N (University of Minnesota Extension, 2021) (Figure 2). However, other research has shown that AL at cooler temperatures can be substantial. Research conducted in Montana for example, has shown that soil temperatures near freezing (29°F – 41°F) can result in AL from surface applied urea and that AL is possible on snow covered soils (Engel et al. 2011). In summary, we learn that lower temperatures may result in AL from surface applied urea, but that this loss increases with an increase in temperature.
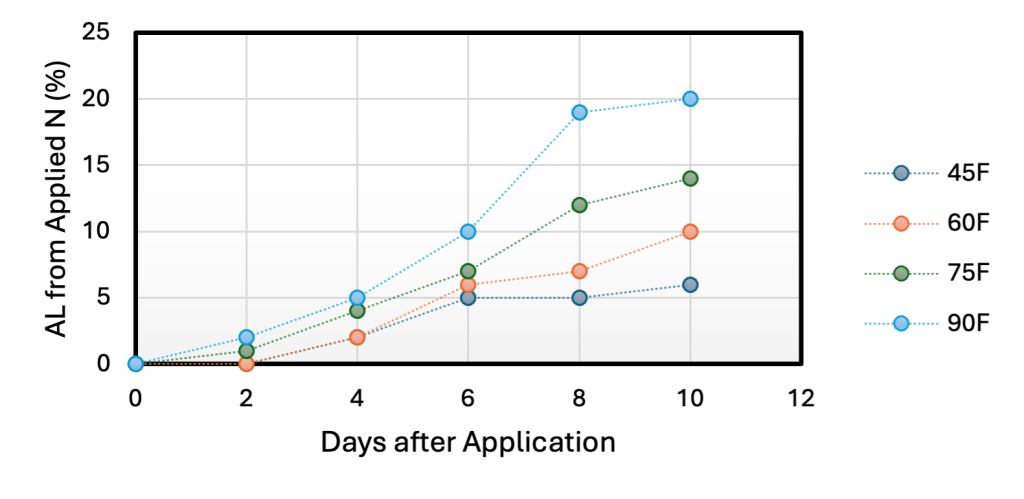
Figure 2. Percentage of applied N lost in ammonia volatilization at different temperatures and days on the surface. Adopted from University of Minnesota Extension (2021).
Another field property that affects AL is crop residue. Urease activity in residue layers can be higher than in the underlying soil. McInnes et al. (1986) found significantly higher urease activity in wheat straw residue compared to the top 0.5 inches of a Muir silt loam. In addition, surface residue can also function as a barrier to the infiltration of urea into soil, while also preventing the underlying soil from drying and therefore maintaining higher rates of AL over a longer period (Hargrove, 1988). The potential for AL thus increases on soils with plant residue to which urea has been surface applied.
Environmental Factors and Ammonia Volatilization
Soil Moisture, Rainfall, Relative Humidity, and Windspeed
Urea must be dissolved before it can be hydrolyzed. Dissolution is when solid urea absorbs water and changes to a liquid form. Urea can absorb water both in the form of liquid or as a vapor. Thus, when urea is applied to soil it might absorb water from the soil to which the urea is applied and from the atmosphere. A review of the literature reveals that the relationship between soil moisture and ammonia volatilization is quite variable due to differences in soil and environmental factors between studies. The general trend that emerges, however, is that when conditions near or at the soil surface remain dry, AL will be insignificant. However, if the soil becomes moist and complete hydrolysis occurs, AL can be substantial especially if diffusion into the soil is minimal and much of the ammonium remains at the soil surface (Ferguson et al. 1984). Consequently, if a rainfall event provides enough moisture for hydrolysis, but not enough for infiltration of urea and is followed by a period of drying, the risk for AL increases. Rainfall or irrigation soon after urea application helps to move urea into the soil where it is more protected and less susceptible to AL. Rainfall’s ability to cause urea infiltration into the soil depends on the magnitude of the rainfall event and the initial soil moisture conditions (Engel et al. 2011). Bundy (2001) noted that a rainfall event of 0.2 to 0.5 inches occurring within 24 hours after application prevents AL.
Relative humidity is the amount of water vapor in the air relative to the maximum amount of water vapor the air can hold at the same temperature. As the relative humidity increases, the potential for absorption of water by urea increases. When the relative humidity exceeds the critical relative humidity, which is the humidity at which urea dissolves, and conditions near the soil surface remain dry, AL increases (Cabrera et al. 2010).
Ammonia loss increases in windy conditions as gaseous molecules at or near the soil surface are rapidly dispersed (Freney et al. 1983). This process is limited by the fact that the supply of ammonia near the soil surface will at some point be depleted, and, as a result, a further increase in windspeed will have no effect on AL.
Managing Urea to Reduce Losses
Source and Placement
There is a difference between N sources when it comes to their susceptibility to AL. Urea as an N source is more susceptible to AL than UAN because the N is 100% urea, while only 50% of N in UAN is urea. One management strategy to reduce AL from urea-based fertilizers, and arguably the most effective, is to incorporate them into the soil which restricts the upward diffusion of ammonia and increases the retention of ammonium on soil particles (Rochette et al. 2013). Urea can be broadcast applied and then incorporated via tillage, or applied in subsurface bands, to reduce AL. Applying urea in a concentrated band places the fertilizer in closer proximity to the seed in comparison to a broadcast application and slows down the conversion and release of plant available N from urea (Wetselaar et al. 1972). Care must be taken not to place urea too close to the seed as urea is very toxic to the seed and may result in reduced yields; a band that is at least 2 inches from the seed is recommended (University of Minnesota Extension, 2021). Rochette et al. (2013) found that compared to surface application of urea, banding resulted in a 12.5% per centimeter reduction in AL.
If incorporation of urea is not an option, an alternative to broadcast surface application is surface banding, which concentrates the urea in a band and reduces its direct exposure to soil and thus urease, thereby reducing the rate of hydrolysis (Hargrove, 1988). In a study conducted in Illinois, an average yield increase of 6.5 bu/ac was found when UAN was dribble banded in comparison to when it was surface applied (Varsa et al. 1999). In a study conducted in Oklahoma, researchers found a numerical, but not statistical, difference in corn yield between surface banding and broadcast application of urea (Oyebiyi et al. 2019).
Urease Inhibitor and Controlled Release Urea
Another way to reduce AL and increase nitrogen recovery is to apply urea with a urease inhibitor. When urease inhibitors are applied with fertilizer, they interfere with the enzymatic activity of urease and therefore hydrolysis (Equation 1). With surface application and other factors that favor AL, urease inhibitors delay hydrolysis hopefully until conditions become less risky for ammonia loss and more favorable for availability and uptake by the crop. Urease inhibitors are commonly added directly to the fertilizer and include brands like Agrotain®, Agrotain Plus®, Nutrisphere-N®, and SuperU®. The urease inhibitor that is considered to be the most widely distributed is N-(n-butyl) thiophosphoric triamide (NBPT), marketed as Agrotain® (Cantarella et al. 2018). By blocking active sites of the urease enzyme, NBPT molecules prevent urea from reacting with urease (Manunza et al. 1999). Although considered an effective technology to reduce AL, positive yield responses due to NBPT are inconsistent. In a meta-analysis by Silva et al. (2017), yield increased by 5.3% in medium textured soils. In coarse-textured soils, however, increases in yield were limited, although the use of NBPT delayed AL and reduced AL from urea by 52%.
Polymer coated urea (PCU) is a product in which each urea granule is coated with a polymer that slows the release of urea into the soil. Marketed under several brand names like ESN® and Duration CR® among others, the advantage of PCU over uncoated urea is that it prevents large amounts of ammonium from becoming available early in the growing season thereby reducing the risk for AL. A study out of Missouri evaluated the effects of urea treated with urease- and nitrification inhibitors, and PCU, collectively called Enhanced Efficiency Fertilizers (EEFs), on AL and grain yield. In this study, Torralbo et al. (2022) found that Duration CR® was the only EEF that produced significantly higher yields than untreated urea across all three years of the study (Table 3). In 2009 and 2010, Duration CR® did not increase yields compared to UAN. Measurements of AL 25 days after application found the lowest losses in the Duration CR®, UAN, and 0N treatments in 2009, while the lowest losses in 2010 were found in the Agrotain®, Duration CR®, ESN®, and 0N treatments. The study found that the PCUs, ESN® and Duration CR®, and urease inhibitor-based fertilizer Agrotain®, were most effective at reducing AL and outperformed the double inhibitor products.
Table 3. Relative corn yield with different urea products with and without enhanced efficiency fertilizer (EEF) in Missouri. Adapted from Torralbo et. al (2022).
2009 | 2010 | 2011 | |
Treatment | Relative Yield (%) | ||
0N | 27 | 12 | 37 |
Urea | 75 | 43 | 62 |
UAN | 90 | 100 | – |
Agrotain* | 80 | 35 | 92 |
Duration** | 100 | 78 | 100 |
ESN** | 81 | 56 | 95 |
Nutrisphere*** | 71 | 33 | 81 |
SuperU*** | – | 77 | 86 |
EEF Mechanisms: *Urease inhibitor, **Polymer-coated urea, ***Urease and nitrification inhibitor.
Summary
Incorporating urea is an effective management strategy to reduce AL from urea, however, it might not always be practical or desirable to do so. Ammonia loss from urea is a complex process that is affected by several soil, environmental, and management variables that interact with each other. In other words, AL is highly variable and difficult to predict. However, we do know some basic principles that should be considered when surface applying urea and urea-based fertilizers:
- AL increases with increasing soil temperatures, with significant losses occurring at soil temperatures > 60°F. To minimize AL, consider applying urea-based fertilizers when soil temperatures are below 60°F.
- Moist soil conditions followed by drying will increase AL from surface-applied urea. Consider timing urea-based fertilizer applications when abundant and well-distributed rainfalls are forecasted or before irrigation. This is especially important on high residue soils.
- Because higher windspeeds result in higher AL, consider applying urea-based fertilizers when wind speeds are relatively low.
- Consider applying UAN instead of urea as a source of N as urea is more susceptible to volatilization.
- Consider applying a urease inhibitor such as NBPT (Agrotain®) with urea or consider applying polymer coated urea (PCU), especially during high AL risk conditions.
- As an alternative to broadcast application of urea, consider surface banding which may reduce AL.
References
DATCP. 2024. Personal communication Department of Agriculture, Trade, and Consumer Protection. Agricultural Resource Management Division-Agrichemical Management Bureau.
Bundy, L. 2001. Managing urea-containing fertilizers. Prepared for Area Fertilizer Dealer Meetings. Soil Science Extension. https://extension.soils.wisc.edu/wp-content/uploads/sites/68/2014/02/ManagingUrea.pdf . Accessed 4/2/2024.
Cabrera, M.L., D.E. Kissel, J.R. Craig, N.P. Qafoku, N. Vaio, J.A. Rema, and L.A. Morris. 2010. Relative humidity controls ammonia loss from urea applied to loblolly pine. Soil Sci. Soc. Am. J. 74: 543-549. https://doi.org/10.2136/SSSAJ2009.0220
Cantarella, H., R. Otto, J. R. Soares, A. G. de Brito Silv. 2018. Agronomic efficiency of NBPT as a urease inhibitor: A review. J. Adv. Res. 13: 19-27. https://doi.org/10.1016/j.jare.2018.05.008.
Engel, R., C. Jones, and R. Wallander. 2011. Ammonia volatilization from urea and mitigation by NBPT following surface application to cold soil. Soil Sci. Soc. Am. J. 75: 2348-2357. https://doi.org/10.2136/SSSAJ2011.0229
Ferguson, R.B., D.E. Kissel, J.K. Koelliker, and W. Basel. 1984. Ammonia volatilization from surface-applied urea: effect of hydrogen ion buffering capacity. Soil Sci. Soc. Am. J. 48:578-582. https://doi.org/10.2136/SSSAJ1984.03615995004800030022X
Freney, J.R., J.R. Simpson, and O.T. 1983. Volatilization of ammonia. In: Freney, J.R., J.R. Simpson (eds) Gaseous loss of nitrogen from plant-soil systems. Developments in Plant and Soil Sciences 9: 1-32 Springer, Dordrecht. https://doi.org/10.1007/978-94-017-1662-8_1
Hargrove, W.L. 1988. Evaluation of ammonia volatilization in the field. J.Prod. Agric. 1: 104-111.
Hendrickson, L.L. 1992. Corn yield response to urease inhibitor NBPT: Five-year summary. J. Prod. Agric. 5: 131-137. https://doi.org/10.2134/jpa1992.0131.
Jones, C.A., R.T. Koenig. J.W. Ellsworth, B.D. Brown, and G.D. Jackson. 2007. Management of urea fertilizer to minimize volatilization. Montana State University Extension. Bozeman, MT.
Manunza, B., S. Deiana, M. Pintore, and C. Gessa. 1999. The binding mechanism of urea, hydroxamic acid and N-(N-butyl)-phosphoric triamide to the urease active site. A comparative molecular dynamics study. Soil Biol. Biochem. 31:789-796. https://doi.org/10.1016/S0038-0717(98)00155-2.
McInnes, K.J., R.B. Ferguson, D.E. Kissel, and E.T. Kanemasu. 1986. Ammonia loss from applications of urea-ammonium nitrate solution to straw residue. Soil. Sci. Soc. Am. J. 50: 969-974.
Oberle, S.L., and L.G. Bundy. 1988. Ammonia volatilization from surface applied N fertilizers: Effects on corn and grass pasture yields and N uptake. Biol. Fert. Soils. 4:185-192. https://doi.org/10.1007/BF00270939
Oyebiyi, F.B., L. Aula, P. Omara, E. Nambi, J. S. Dhillon, and W.R. Raun. 2019. Maize (Zeamays L.) grain yield response to methods of nitrogen fertilization, Comm. Soil Sci. Plant Anal., 50: 2694-2700. https://doi.org/10.1080/00103624.2019.1670837
Pan, B., S.K. Lam, A. Mosier, Y. Luo, and D. Chen. 2016. Ammonia volatilization from synthetic fertilizers and its mitigation strategies: A global synthesis. Agric. Ecosyst. Environ. 232: 283-289. https://doi.org/10.1016/j.agee.2016.08.019.
Rochette, P., D.A. Angers, M.H. Chantigny, M.O. Gasser, J.D. MacDonald, D.E. Pelster, and N. Bertrand. 2013. Ammonia volatilization and nitrogen retention: How deep to incorporate urea? J. Environ. Qual. 42: 1635–1642. https://doi.org/10.2134/jeq2013.05.0192.
Silva, A.G.B., C.H. Sequeira, R.A. Sermarini, and R. Otto. 2017. Urease inhibitor NBPT on ammonia volatilization and crop productivity: A meta-analysis. Agron. J. 109:1-13. https://doi.org/10.2134/agronj2016.04.0200
Torralbo, F., D. Boardman, J.H. Houx, and F.B. Fritschi. 2022. Distinct enhanced efficiency urea fertilizers differentially influence ammonia volatilization losses and maize yield. Plant Soil 475: 551–563. https://doi.org/10.1007/s11104-022-05387-4
University of Minnesota Extension. 2021. Fertilizer Urea. Available at: https://extension.umn.edu/nitrogen/fertilizer-urea . Accessed 5/1/2024.
Varsa, E.C., S.A. Ebelhar, P.R. Eberle, E. Gerhard, and T. Wyciskalla. 1999. An evaluation of urease inhibitor technology as a nitrogen management tool in no-till corn and wheat production: Agronomics and economics. Illinois Fertilizer Conference Proceedings, 1999.
Wetselaar, R., J.B. Passioura, B.R. Singh. 1972. Consequences of banding nitrogen fertilizers in soil. Plant Soil 36: 159-175.